The Epigenetic code and brain development
Epigenetics has been a hot topic in molecular biology for several years and it´s fascinating to see how it is now trending in general news as well. I was reminded of this fact when hearing Fatimah Jackson speak at the American Museum of Natural History´s recent SciCafe. So what is epigenetics? First of all it´s not as simple as the genetic code!
The name is derived from epi- (Greek: επί- over, outside of, around) combined with genetics, literally meaning being “over genetics”. Epigenetics is the study of heritable changes in gene activity which are NOT caused by changes in the DNA sequence. While the idea that factors other than changes in DNA can affect development was discussed almost a century ago (called “epigenesist” by CH Waddington), epigenetics is now more often considered to be changes to DNA that don’t involve changes in the DNA sequence itself. Moreover the term epigenetics can also be used to describe the study of stable, long-term alterations in the transcriptional potential of a cell that are not necessarily passed on to the next generation. Though this last point is contentious – some scientists believe the definition of epigenetics is modifications to DNA that are passed onto the next generation of either daughter cells (mitosis) or germ cells (meiosis).
So unlike simple genetics, where mutations affect the genotype by changing letters of the DNA alphabet (the four nitrogenous bases adenine (A), guanine (G), cytosine (C), and thymine (T)), epigenetic changes that cause changes in gene expression have other roots.
So if the DNA is not changed, what is changed instead? Generally this involves chemical modifications around DNA that cause gene expression to be changed, most often silenced. These modifications can act as an extra layer of information and, in the brain, are thought to play an important role in learning and memory, as well as in age-related cognitive decline.
The results of a study by researchers at the Salk Institute for Biological Studies published in Science show that the landscape of DNA methylation – a particular type of epigenetic modification that adds a methyl group (CH3) – is highly variable in brain cells during certain developmental stages. These new findings help us understand how information in the DNA of brain cells is controlled from early fetal development to adulthood.
With humans having exceptionally complex and large brains (larger than any other mammal in relation to body size) it is clear that building and shaping a healthy brain is the product of a long process of development. We know that the front-most part of our brain (the prefrontal cortex) for example is a critical part of the executive system, which refers to planning, reasoning, and judgment. The brain accomplishes all of this through the interaction of specialized cells such as neurons and glia, the brain’s communication specialists. We know that these cells have distinct functions.
But now epigenetics tells us what gives these cells their individual identities! It all depends on how each cell expresses the genetic code. And this how is done by epigenetic modifications, fine-tuning which genes are turned on or off without changing the DNA sequence, and thus subsequently helping to distinguishing different cell types.
The Salk scientists found that the patterns of DNA methylation undergo dynamic rearrangements in the frontal cortex of mouse and human brains during a time of development when synapses, or connections between nerve cells, grow rapidly. By comparing the exact sites of DNA methylation throughout the genome in brains from infants through adults, the researchers noticed that one form of DNA methylation can be found in neurons and glia from birth. However, a second form of DNA methylation that is almost exclusive to neurons accumulates as the brain matures, becoming the most prevalent form of DNA methylation in adult human neurons.
Teachers and child development experts have long known about natural breakage points in a child’s development. These new results can help us to understand how those points occur as the intricate DNA landscape of brain cells develops during the key stages of childhood.
What is the mechanism of DNA methylation? As mentioned above, the genetic code in DNA is made up of four nitrogenous bases A, T, C, and G. DNA methylation typically occurs at so-called “CpG sites,” where C (cytosine) sits next to G (guanine) in the DNA alphabet. Interestingly about 80–90% of CpG sites are methylated in human DNA. Moreover, in human embryonic stem cells and induced pluripotent stem cells, a type of artificially derived stem cell, DNA methylation can also occur when G does not follow C, called “non-CG methylation.” Originally, scientists thought that this type of methylation disappeared when stem cells differentiate into specific tissue-types. This latest study found this is not the case in the brain, because non-CG methylation appears after cells differentiate, and they usually differentiate during childhood and adolescence when the brain is maturing. What this finding underlines is that at the time the neural circuits of the brain mature, a parallel process of large-scale reconfiguration of the neural epigenome takes place.
The study also included the first comprehensive maps of how DNA methylation patterns change in the mouse and human brain during development (see insert). Future research can explore how changes in methylation patterns may be linked to human diseases, including psychiatric disorders like schizophrenia, depression, and bipolar disorder.
—————-
Further reading:
You can even get a quick PowerPoint podcast on the research!
https://dl.dropboxusercontent.com/u/1421283/Epigenome_Movie.mov
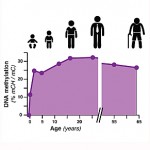
The first comprehensive maps of epigenetic changes in the brain known as "DNA methylation," a chemical modification of a cell's DNA that can act as an extra layer of information in the genome. Credit: Lister et al, 2013.
Print article | This entry was posted by Christine Marizzi on January 21, 2014 at 11:34 am, and is filed under G2C Online. Follow any responses to this post through RSS 2.0. You can leave a response or trackback from your own site. |